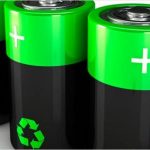
Wednesday, July 04, 2018 by Edsel Cook
http://www.futuresciencenews.com/2018-07-04-physicists-are-one-step-closer-to-developing-optoelectronic-devices-that-are-smaller.html
Swiss physicists from ETH Zurich have succeeded in measuring electron response in a semiconductor at the attosecond (one quintillionth of a second) timescale. This startling breakthrough is expected to spur the development of optoelectronic devices that can operate in the petahertz level, reported Science Daily.
The previous maximum resolution of electron response was below a femtosecond (one quadrillionth of a second).
Optoelectronic devices are a type of apparatus that rely on the use of light to stimulate electrons. Examples include photodiodes, photovoltaic cells, light-emitting diodes (LEDs), and fiber-optic wire.
These devices see a wide variety of applications in modern technology. Cameras, for instance, use photodiodes to take photographs while the photovoltaic cells in solar panels turn sunlight into electrical energy.
The ability to measure the excitement of electrons in semiconductors at smaller time units will enable greater accuracy and faster speeds in all kinds of optoelectronic devices. (Related: Engineers develop breakthrough method of combining solar panels and light-emitting devices.)
The ETH Zurich research team achieved this result using gallium arsenide (GaAs). An important compound that sees wide use in electronic circuits, GaAs is a narrow-band-gap semiconductor with a smaller band-gap than silicon.
A band-gap is an energy range in which no electron states can exist. The smaller the band-gap, the better the material is at conducting electrons. Semiconductors like silicon usually have moderate band-gaps, but gallium arsenide has a smaller one than silicon.
Laser light is used to excite the electrons of a semiconductor like GaAs. These energized electrons jump from the valence to the conduction band. What results is “inter-band transition,” where charge carriers carry an electrical current through electronic parts, enabling the function of electronic circuits.
Furthermore, laser light can also be used to speed up charge-carrying electronics within individual conduction bands. This is because laser light has a strong electric field, which enables intra-band motion.
Researchers were not sure which mechanism takes precedence in generating the electrons’ response to an intense laser pulse. Neither is it clear how the relationship between inter-band transition and intra-band motion affects the carrier injection into the conduction band.
The ETH research group led by Fabian Schlaepfer employed a mix of ultra-fast flash spectroscopy and first-principles calculations based on the laws of quantum mechanics. They were able to observe inter-band transition and intra-band motion for the first time at the attosecond timescale.
They published their findings in the online journal Nature Physics.
Schlaepfer and his colleagues discovered that intra-band motion plays an important role in electron excitement. The mechanism enables a much large number of electrons to make the jump into the conduction band.
By itself, intra-band motion is unable to produce charge carriers in the conduction band. The realization that it can complement the effect of inter-band transition makes this discovery of particular interest and importance.
Future electronics and optoelectronic devices are following the trend of decreasing the size of the gadget while also increasing its capabilities to match bigger, older equivalents.
For example, many smartphones today have more computing power than desktop personal computers from the 1990s.
As devices grow smaller, the relevant electric fields increase in strength and the dynamics speed up. Even femtosecond-level observation of electron dynamics – a capability developed over the past decade – will no longer suffice.
The ability to observe light-induced electron dynamics in a semiconductor on the attosecond timescale is invaluable. It will possibly enable the development of even smaller optoelectronic devices that can leverage previously mysterious mechanisms – such as intra-band motion – to squeeze out even more performance.
For more updates on optoelectronics, visit Scientific.news.
Sources include: